Механизмы клеточной устойчивости к ингибитору трансляции микроцину С и родственным соединениям
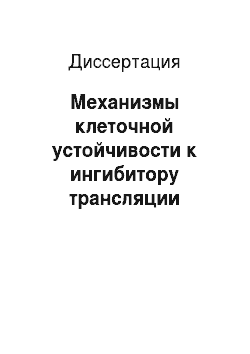
Fersht, A.R. and C. Dingwall, Cysteinyl-tRNA synthetase from Escherichia coli does not need an editing mechanism to reject serine and alanine. High binding energy of small groups in specific molecular interactions. Biochemistry, 1979.18(7): p. 1245−9. Gentry, D.R., et al., Variable Sensitivity to Bacterial Methionyl-tRNA Synthetase Inhibitors Reveals Subpopulations of Streptococcus pneumoniae… Читать ещё >
Содержание
- СПИСОК СОКРАЩЕНИЙ
- ОБЩАЯ ХАРАКТЕРИСТИКА РАБОТЫ Актуальность работы
- Цели и задачи новизна и научная значимость работы
- Публикации и апробация работы
- ЛИТЕРАТУРНЫЙ ОБЗОР
Механизмы клеточной устойчивости к ингибитору трансляции микроцину С и родственным соединениям (реферат, курсовая, диплом, контрольная)
Выводы.
1) MccF является сериновой пептидазой, которая обеспечивает устойчивость клетки к МсС за счет гидролиза амидной связи между пептидной и нуклеотидной частями антибиотика. Кроме МсС MccF гидролизует aaSA с полярными отрицательно заряженными аминоацильными группами.
2) Определена структура MccF и выяснена молекулярная природа узнавания субстрата. Специфичность MccF к аденилированным субстратам опосредована остатком ароматической аминокислоты, расположенном в петле между двумя доменами белка.
3) В S66 семействе пептидаз найдена группа гомологов MccF, которые содержат междоменную петлю с консервативным ароматическим остатком. Показано, что некоторые представители этой группы гидролизуют МсС и aaSA. Гомолог MccF из В. anthracis обладает сходной с MccF из Е. coli структурой и механизмом действия и в физиологических условиях защищает клетки от химического аналога МсС.
Публикации в журналах:
Tikhonov A, Kazakov Т, Semenova Е, Serebryakova М, Vondenhoff G, Van Aerschot A, Reader JS, Govorun VM, Severinov K. The mechanism of Microcin С resistance provided by the MccF peptidase. Journal of Biological Chemistry. 2010 Dec 3- 285(49): 37 944−52.
Agarwal V, Tikhonov A, Metlytskaya A, Severinov K, and Nair S. Structure and function of a serine carboxypeptidase adapted for degradation of the protein synthesis antibiotic microcin CI. Proceedings of the National Academy of Sciences USA. 2012 Mar 20- 109(12): 4425−30.
Nocek BTikhonov ABabnigg GMinyi GuZhou MMakarova KSVondenhoff GAerschot AVKwon KAnderson WFSeverinov KJoachimiak A. Structural and functional characterization of microcin С resistance peptidase MccF from Bacillus anthracis. Journal of Molecular Biology. 2012 Jul 20- 420(4−5): 366−83.
Заключение
.
В данной работе с помощью разнообразных методов был подробно изучен механизм действия МссР, за счет которого клетки приобретают устойчивость к МсС. Полученные нами результаты расширяют представления о спектре субстратной специфичности и биохимии сериновых пептидаз. Кроме того, мы обнаружили большое количество гомологов МссР среди бактерий, и показали, что некоторые из найденных гомологов способны гидролизовать субстраты МссР. Эти результаты косвенно указывают на значительное распространение токсичных негидролизуемых аминоацил-аденилатов в бактериальных сообществах. Изучение физиологических функций найденных белков представляется интересной научной задачей. !!" «!!» .
1. Klevens, R.M., et al., Estimating health care-associated infections and deaths in U.S. hospitals, 2002. Public Health Rep, 2007.122(2): p. 160−6.
2. Kong, K.F., L. Schneper, and K. Mathee, Beta-lactam antibiotics: from antibiosis to resistance and bacteriology. APMIS, 2010. 118(1): p. 1−36.
3. Nikaido, H., Multidrug resistance in bacteria. Annu Rev Biochem, 2009. 78: p. 119−46.
4. Auerbach, T., A. Bashan, and A. Yonath, Ribosomal antibiotics: structural basis for resistance, synergism and selectivity. Trends Biotechnol, 2004. 22(11): p. 570−6.
5. Drlica, K., et al., Quinolone-mediated bacterial death. Antimicrob Agents Chemother, 2008. 52(2): p. 385−92.
6. Poehlsgaard, J. and S. Douthwaite, The bacterial ribosome as a target for antibiotics. Nat Rev Microbiol, 2005. 3(11): p. 870−81.
7. Hurdle, J.G., A.J. O’Neill, and I. Chopra, Prospects for Aminoacyl-tRNA Synthetase Inhibitors as New Antimicrobial Agents. Antimicrob Agents Chemother, 2005. 49(12): p. 4821−4833.
8. Ibba, M. and D. Soil, Aminoacyl-tRNA synthesis. Annu Rev Biochem, 2000. 69: p. 617−50.
9. Hong, K.W., et al., Transfer RNA-dependent cognate amino acid recognition by an aminoacyl-tRNA synthetase. EMBO J, 1996. 15(8): p. 1983;91.
10. Ruff, M., et al., Class II aminoacyl transfer RNA synthetases: crystal structure of yeast aspartyl-tRNA synthetase complexed with tRNA (Asp). Science, 1991. 252(5013): p. 1682−9.
11. Cusack, S., et al., A second class of synthetase structure revealed by X-ray analysis of Escherichia coli seryl-tRNA synthetase at 2.5 A. Nature, 1990. 347(6290): p. 249−55.
12. Eriani, G., et al., Partition of tRNA synthetases into two classes based on mutually exclusive sets of sequence motifs. Nature, 1990. 347(6289): p. 203−6.
13. Burbaum, J.J. and P. Schimmel, Structural relationships and the classification of aminoacyl-tRNA synthetases. J Biol Chem, 1991. 266(26): p. 16 965−8.
14. Arnez, J.G. and D. Moras, Structural and functional considerations of the aminoacylation reaction. Trends Biochem Sci, 1997. 22(6): p. 211−6.
15. Beuning, P.J., et al., Specific atomic groups and RNA helix geometry in acceptor stem recognition by a tRNA synthetase. Proc Natl Acad Sci USA, 1997. 94(19): p. 10 150−4.
16. Ochsner, U.A., et al., Aminoacyl-tRNA synthetases: essential and still promising targets for new anti-infective agents. Expert Opin Investig Drugs, 2007. 16(5): p. 573−93.
17. Giege, R., M. Sissler, and C. Florentz, Universal rules and idiosyncratic features in tRNA identity. Nucleic Acids Res, 1998. 26(22): p. 5017−35.
18. Giege, R., J.D. Puglisi, and C. Florentz, tRNA structure and aminoacylation efficiency. Prog Nucleic Acid Res Mol Biol, 1993. 45: p. 129−206.
19. Ling, J., N. Reynolds, and M. Ibba, Aminoacyl-tRNA synthesis and translational quality control. Annu Rev Microbiol, 2009. 63: p. 61−78.
20. Nicholas, H.B., Jr. and W.H. McClain, Searching tRNA sequences for relatedness to aminoacyl-tRNA synthetase families. J Mol Evol, 1995. 40(5): p. 482−6.
21. Ibba, M., et al., Transfer RNA identity contributes to transition state stabilization during aminoacyl-tRNA synthesis. Nucleic Acids Res, 1999. 27(18): p. 3631−7.
22. Fersht, A.R. and C. Dingwall, Cysteinyl-tRNA synthetase from Escherichia coli does not need an editing mechanism to reject serine and alanine. High binding energy of small groups in specific molecular interactions. Biochemistry, 1979.18(7): p. 1245−9.
23. Schmidt, E. and P. Schimmel, Mutational isolation of a sieve for editing in a transfer RNA synthetase. Science, 1994. 264(5156): p. 265−7.
24. Ibba, M. and D. Soil, Quality control mechanisms during translation. Science, 1999. 286(5446): p. 1893−7.
25. Farrow, M.A., B.E. Nordin, and P. Schimmel, Nucleotide determinants for tRNA-dependent amino acid discrimination by a class I tRNA synthetase. Biochemistry, 1999. 38(51): p. 16 898−903.
26. Eldred, E.W. and P.R. Schimmel, Rapid deacylation by isoleucyl transfer ribonucleic acid synthetase of isoleucine-specific transfer ribonucleic acid aminoacylated with valine. J Biol Chem, 1972. 247(9): p. 2961−4.
27. Jakubowski, H. and E. Goldman, Editing of errors in selection of amino acids for protein synthesis. Microbiol Rev, 1992. 56(3): p. 412−29.
28. Jakubowski, H., Aminoacyl thioester chemistry of class II aminoacyl-tRNA synthetases. Biochemistry, 1997. 36(37): p. 11 077−85.
29. Calendar, R. and P. Berg, D-Tyrosyl RNA: formation, hydrolysis and utilization for protein synthesis. J Mol Biol, 1967. 26(1): p. 39−54.
30. Ferri-Fioni, M.L., et al., Identification in archaea of a novel D-Tyr-tRNATyr deacylase. J Biol Chem, 2006. 281(37): p. 27 575−85.
31. Wydau, S., et al., GEK1, a gene product of Arabidopsis thaliana involved in ethanol tolerance, is a D-aminoacyl-tRNA deacylase. Nucleic Acids Res, 2007. 35(3): p. 930−8.
32. An, S. and K. Musier-Forsyth, Trans-editing of Cys-tRNAPro by Haemophilus influenzae YbaK protein. J Biol Chem, 2004. 279(41): p. 42 359−62.
33. Ahel, I., et al., Trans-editing of mischarged tRNAs. Proc Natl Acad Sci USA, 2003. 100(26): p. 15 422−7.
34. Korencic, D., et al., A freestanding proofreading domain is required for protein synthesis quality control in Archaea. Proc Natl Acad Sci USA, 2004. 101(28): p. 10 260−5.
35. Wilcox, M., Gamma-glutamyl phosphate attached to glutamine-specific tRNA. A precursor of glutaminyl-tRNA in Bacillus subtilis. Eur J Biochem, 1969. 11(3): p. 405−12.
36. Schon, A., H. Hottinger, and D. Soil, Misaminoacylation and trans amidation are required for protein biosynthesis in Lactobacillus bulgaricus. Biochimie, 1988. 70(3): p. 391−4.
37. Schon, A., et al., Protein biosynthesis in organelles requires misaminoacylation of tRNA. Nature, 1988. 331(6152): p. 187−90.
38. Stanzel, M., A. Schon, and M. Sprinzl, Discrimination against misacylated tRNA by chloroplast elongation factor Tu. Eur J Biochem, 1994. 219(1−2): p. 435−9.
39. Curnow, A.W., M. Ibba, and D. Soli, tRNA-dependent asparagine formation. Nature, 1996. 382(6592): p. 589−90.
40. Schulze, J.O., et al., Crystal structure of a non-discriminating glutamyl-tRNA synthetase. J Mol Biol, 2006. 361(5): p. 888−97.
41. Withey, J.H. and D.I. Friedman, A salvage pathway for protein structures: tmRNA and transtranslation. Annu Rev Microbiol, 2003. 57: p. 101−23.
42. Meinnel, T., Y. Mechulam, and S. Blanquet, Methionine as translation start signal: a review of the enzymes of the pathway in Escherichia coli. Biochimie, 1993. 75(12): p. 1061−75.
43. Amberg, R., et al., Selenocysteine synthesis in mammalia: an identity switch from tRNA (Ser) to tRNA (Sec). J Mol Biol, 1996. 263(1): p. 8−19.
44. Putzer, H., et al., Aminoacyl-tRNA synthetase gene regulation in Bacillus subtilis: induction, repression and growth-rate regulation. Mol Microbiol, 1995.16(4): p. 709−18.
45. Sankaranarayanan, R., et al., The structure of threonyl-tRNA synthetase-tRNA (Thr) complex enlightens its repressor activity and reveals an essential zinc ion in the active site. Cell, 1999. 97(3): p. 371−81.
46. Rho, S.B., T.L. Lincecum, Jr., and S.A. Martinis, An inserted region of leucyl-tRNA synthetase plays a critical role in group I intron splicing. EMBO J, 2002. 21(24): p. 6874−81.
47. Wakasugi, K. and P. Schimmel, Two distinct cytokines released from a human aminoacyl-tRNA synthetase. Science, 1999. 284(5411): p. 147−51.
48. Chain, E.B. and G. Mellows, Pseudomonic acid. Part 1. The structure of pseudomonic acid A, a novel antibiotic produced by Pseudomonas fluorescens. J Chem Soc Perkin 1, 1977(3): p. 294−309.
49. Hughes, J. and G. Mellows, Inhibition of isoleucyl-transfer ribonucleic acid synthetase in Escherichia coli by pseudomonic acid. Biochem J, 1978. 176(1): p. 305−18.
50. Kim, S., et al., Aminoacyl-tRNA synthetases and their inhibitors as a novel family of antibiotics. Appl Microbiol Biotechnol, 2003. 61(4): p. 278−288.
51. Hurdle, J.G., et al., Analysis of mupirocin resistance and fitness in Staphylococcus aureus by molecular genetic and structural modeling techniques. Antimicrob Agents Chemother, 2004. 48(11): p. 4366−76.
52. Sutherland, R., et al., Antibacterial activity of mupirocin (pseudomonic acid), a new antibiotic for topical use. Antimicrob Agents Chemother, 1985. 27(4): p. 495−498.
53. Cookson, B.D., The emergence of mupirocin resistance: a challenge to infection control and antibiotic prescribing practice. J Antimicrob Chemother, 1998. 41(1): p. 11−8.
54. Davey, P., Eradication of nasal carriage of Staphylococcus aureus~is it cost-effective? J Hosp Infect, 1998. 40 Suppl B: p. S31−7.
55. Fujimura, S. and A. Watanabe, Survey of highand low-level mupirocin-resistant strains of methicillin-resistant Staphylococcus aureus in 15 Japanese hospitals. Chemotherapy, 2003. 49(1−2): p. 36−8.
56. Gilbart, J., C.R. Perry, and B. Slocombe, High-level mupirocin resistance in Staphylococcus aureus: evidence for two distinct isoleucyl-tRNA synthetases. Antimicrob Agents Chemother, 1993. 37(1): p. 32−8.
57. Brown, J.R., et al., Horizontal transfer of drug-resistant aminoacyl-transfer-RNA synthetases of anthrax and Gram-positive pathogens. EMBO Rep, 2003. 4(7): p. 692−8.
58. Beaulieu, D. and K.A. Ohemeng, Patents on bacterial tRNA synthetase inhibitors: January 1996 to March 1999. Expert Opinion on Therapeutic Patents, 1999. 9(8): p. 1021−1028.
59. Kanamaru, T., et al., In vitro and in vivo antibacterial activities of TAK-083, an agent for treatment of Helicobacter pylori infection. Antimicrob Agents Chemother, 2001. 45(9): p. 2455−9.
60. Werner, R.G., Uptake of indolmycin in gram-positive bacteria. Antimicrob Agents Chemother, 1980.18(6): p. 858−62.
61. Werner, R.G. and W. Reuter, Interaction of indolmycin in the metabolism of tryptophan in rat liver. Arzneimittelforschung, 1979. 29(1): p. 59−63.
62. Hurdle, J.G., A.J. O’Neill, and I. Chopra, Anti-staphylococcal activity of indolmycin, a potential topical agent for control of staphylococcal infections. J Antimicrob Chemother, 2004. 54(2): p. 549−52.
63. Brown, M.J., et al., The antimicrobial natural product chuangxinmycin and some synthetic analogues are potent and selective inhibitors of bacterial tryptophanyl tRNA synthetase. Bioorg Med Chem Lett, 2002.12(21): p. 3171−4.
64. Hutter, R., et al., Metabolic products of microorganisms. 51. On the mechanism of action of borrelidin-inhibition of the threonine incorporation in sRNAJ. Biochem Z, 1966. 344(2): p. 190−6.
65. Otoguro, K., et al., In vitro and in vivo antimalarial activities of a non-glycosidic 18-membered macrolide antibiotic, borrelidin, against drug-resistant strains of Plasmodia. J Antibiot (Tokyo), 2003. 56(8): p. 727−9.
66. Habibi, D., et al., Borrelidin, a small molecule nitrile-containing macrolide inhibitor of threonyl-tRNA synthetase, is a potent inducer of apoptosis in acute lymphoblastic leukemia. Invest New Drugs, 2011.
67. Ruan, B., et al., A unique hydrophobic cluster near the active site contributes to differences in borrelidin inhibition among threonyl-tRNA synthetases. J Biol Chem, 2005. 280(1): p. 571−7.
68. Paetz, W. and G. Nass, Biochemical and immunological characterization of threonyl-tRNA synthetase of two borrelidin-resistant mutants of Escherichia coli K12. Eur J Biochem, 1973. 35(2): p. 331−7.
69. Oki, T., et al., Cispentacin, a new antifungal antibiotic. II. In vitro and in vivo antifungal activities. J Antibiot (Tokyo), 1989. 42(12): p. 1756−62.
70. Konishi, M., et al., Cispentacin, a new antifungal antibiotic. I. Production, isolation, physico-chemical properties and structure. J Antibiot (Tokyo), 1989. 42(12): p. 1749−55.
71. Snipes, C.E., C.-J. Chang, and H.G. Floss, Biosynthesis of the antibiotic granaticin. J Am Chem Soc, 1979.101(3): p. 701−706.
72. Chang, C.J., et al., Identity of the antitumor antibiotic litmomycin with granaticin A. J Antibiot (Tokyo), 1975. 28(2): p. 156.
73. Katagiri, K., et al., A new antibiotic. Furanomycin, an isoleucine antagonist. J Med Chem, 1967. 10(6): p. 1149−54.
74. Kohno, T., et al., Nonprotein amino acid furanomycin, unlike isoleucine in chemical structure, is charged to isoleucine tRNA by isoleucyl-tRNA synthetase and incorporated into protein. Journal of Biological Chemistry, 1990. 265(12): p. 6931−5.
75. Larsen, T.O., A. Svendsen, and J. Smedsgaard, Biochemical characterization of ochratoxin A-producing strains of the genus Penicillium. Appl Environ Microbiol, 2001. 67(8): p. 3630−5.
76. Moss, M.O., Mycotoxin review 1. Aspergillus and Penicillium. Mycologist, 2002. 16(03): p. 116−119.
77. Dirhaimer, G., Creppy, E.E., Mechanism of action ochratoxin A. IARC Sci Publ, 1991. 115: p. 171−86.
78. Bennett, I., et al., Synthesis and antibacterial properties of beta-diketone acrylate bioisosteres ofpseudomonic acid A. Bioorg Med Chem Lett, 1999. 9(13): p. 1847−52.
79. Broom, N.J.P., et al., The Chemistry of Pseudomonic Acid. 17. Dual-Action C-l Oxazole Derivatives of Pseudomonic Acid Having an Extended Spectrum of Antibacterial Activityf. J Med Chem, 1996. 39(18): p. 3596−3600.
80. Barker, J.J., Antibacterial drug discovery and structure-based design. Drug Discov Today, 2006.11(9−10): p. 391−404.
81. Ziegelbauer, K., P. Babczinski, and W. Schonfeld, Molecular mode of action of the antifungal beta-amino acid BAY 10−8888. Antimicrob Agents Chemother, 1998. 42(9): p. 2197−205.
82. Rock, F.L., et al., An Antifungal Agent Inhibits an Aminoacyl-tRNA Synthetase by Trapping tRNA in the Editing Site. Science, 2007. 316(5832): p. 1759−1761.
83. Nare, B., et al., Discovery of Novel Orally Bioavailable Oxaborole 6-Carboxamides That Demonstrate Cure in a Murine Model of Late-Stage Central Nervous System African Trypanosomiasis. Antimicrob Agents Chemother, 2010. 54(10): p. 4379−4388.
84. Jarvest, R.L., et al., Nanomolar Inhibitors of Staphylococcus aureus Methionyl tRNA Synthetase with Potent Antibacterial Activity against Gram-Positive Pathogens. J Med Chem, 2002. 45(10): p. 1959;1962.
85. Critchley, I.A., et al., Antibacterial Activity of REP8839, a New Antibiotic for Topical Use. Antimicrob Agents Chemother, 2005. 49(10): p. 4247−4252.
86. Kim, S.Y. and J. Lee, 3-D-QSAR study and molecular docking of methionyl-tRNA synthetase inhibitors. Bioorganic &Medicinal Chemistry, 2003.11(24): p. 5325−5331.
87. Gentry, D.R., et al., Variable Sensitivity to Bacterial Methionyl-tRNA Synthetase Inhibitors Reveals Subpopulations of Streptococcus pneumoniae with Two Distinct Methionyl-tRNA Synthetase Genes. Antimicrob Agents Chemother, 2003. 47(6): p. 1784−1789.
88. Beyer, D., et al., New Class of Bacterial Phenylalanyl-tRNA Synthetase Inhibitors with High Potency and Broad-Spectrum Activity. Antimicrob Agents Chemother, 2004. 48(2): p. 525−532.
89. Isono, K., et al., Ascamycin and dealanylascamycin, nucleoside antibiotics from Streptomyces sp. J Antibiot (Tokyo), 1984. 37(6): p. 670−2.
90. Osada, H. and K. Isono, Mechanism of action and selective toxicity of ascamycin, a nucleoside antibiotic. Antimicrob Agents Chemother, 1985. 27(2): p. 230−3.
91. Osada, H. and K. Isono, Purification and characterization of ascamycin-hydrolysing aminopeptidase from Xanthomonas citri. Biochem J, 1986. 233(2): p. 459−63.
92. Uramoto, M., et al., Isolation and characterization of phosmidosine. A new antifungal nucleotide antibiotic. J Antibiot (Tokyo), 1991. 44(4): p. 375−81.
93. Phillips, D.R., et al., Structure of the antifungal nucleotide antibiotic phosmidosine. The Journal of Organic Chemistry, 1993. 58(4): p. 854−859.
94. Sekine, M., et al., Synthesis of Chemically Stabilized Phosmidosine Analogues and the Structure-Activity Relationship of Phosmidosine. The Journal of Organic Chemistry, 2003. 69(2): p. 314−326.
95. Gause, G.F., Recent studies on albomycin, a new antibiotic. Br Med J, 1955. 2(4949): p. 11 779.
96. Benz, G., et al., Constitution of the Deferriform of the Albomycins SI, 62 and e. Angewandte Chemie International Edition in English, 1982. 21(7): p. 527−528.
97. Pramanik, A., et al., Albomycin is an effective antibiotic, as exemplified with Yersinia enterocolitica and Streptococcus pneumoniae. International Journal of Medical Microbiology, 2007. 297(6): p. 459−469.
98. Braun, V., et al., Sideromycins: tools and antibiotics. Biometals, 2009. 22(1): p. 3−13.
99. Braun, V., et al., Intracellular activation of albomycin in Escherichia coli and Salmonella typhimurium. J Bacterid, 1983. 156(1): p. 308−315.
100. Stefanska, A.L., et al., A potent seryl tRNA synthetase inhibitor SB-217 452 isolated from a Streptomyces species. J Antibiot (Tokyo), 2000. 53(12): p. 1346−53.
101. Tate, M.E., et al., Adenine N6-substituent of agrocin 84 determines its bacteriocin-like specificity. Nature, 1979. 280(5724): p. 697−9.
102. Gelvin, S.B., AGROBACTERIUM AND PLANT GENES INVOLVED IN T-DNA TRANSFER AND INTEGRATION. Annual Review of Plant Physiology and Plant Molecular Biology, 2000. 51(1): p. 223−256.
103. Kim, H. and S.K. Farrand, Characterization of the acc operon from the nopaline-type Ti plasmidpTiC58, which encodes utilization of agrocinopines A and B and susceptibility to agrocin 84. J Bacterid, 1997. 179(23): p. 7559−72.
104. Ellis, J.G. and P.J. Murphy, Four new opines from crown gall tumours —Their detection and properties. Molecular and General Genetics MGG, 1981.181(1): p. 36−43.
105. MURPHY, P.J. and W.P. ROBERTS, A Basis for Agrocin 84 Sensitivity in Agrobacterium radiobacter. Journal of General Microbiology, 1979.114(1): p. 207−213.
106. Reader, J.S., et al., Major Biocontrol of Plant Tumors Targets tRNA Synthetase. Science, 2005. 309(5740): p. 1533.
107. Penyalver, R., B. Vicedo, and M.M. Lopez, Use of the Genetically Engineered Agrobacterium Strain K1026 for Biological Control of Crown Gall. European Journal of Plant Pathology, 2000. 106(9): p. 801−810.
108. Garcia-Bustos, J.F., N. Pezzi, and E. Mendez, Structure and mode of action of microcin 7, an antibacterial peptide produced by Escherichia coli. Antimicrob Agents Chemother, 1985. 27(5): p. 791−797.
109. Cursino, L., et al., Exoproducts of the Escherichia coli strain H22 inhibiting some enteric pathogens both in vitro and in vivo. Journal of Applied Microbiology, 2006.100(4): p. 821−829.
110. Kurepina, N.E., et al., Cloning and mapping of the genetic determinants for microcin C51 production and immunity. Molecular and General Genetics MGG, 1993. 241(5): p. 700−706.
111. Guijarro, J.I., et al., Chemical Structure and Translation Inhibition Studies of the Antibiotic Microcin C7. Journal of Biological Chemistry, 1995. 270(40): p. 23 520−23 532.
112. Gonzalez-Pastor, J.E., et al., Structure and organization of plasmid genes required to produce the translation inhibitor microcin C7. J Bacteriol, 1995. 177(24): p. 7131−40.
113. Smajs, D., et al., Complete sequence of low-copy-number plasmid MccC7-H22 of probiotic Escherichia coli H22 and the prevalence of mcc genes among human E. coli. Plasmid, 2008. 59(1): p. 1−10.
114. Fomenko, D.E., et al., Microcin C51 plasmid genes: possible source of horizontal gene transfer. Antimicrob Agents Chemother, 2003. 47(9): p. 2868−74.'.
115. Gonzalez-Pastor, J.E., J.L. San Millan, and F. Moreno, The smallest known gene. Nature, 1994. 369(6478): p. 281.
116. Roush, R.F., et al., Maturation of an Escherichia coli Ribosomal Peptide Antibiotic by ATP-Consuming N-P Bond Formation in Microcin C7. J Am Chem Soc, 2008. 130(11): p. 3603−3609.
117. Metlitskaya, A., et al., Maturation of the Translation Inhibitor Microcin C. J Bacteriol, 2009. 191(7): p. 2380−2387.
118. Regni, C.A., et al., How the MccB bacterial ancestor of ubiquitin El initiates biosynthesis of the microcin C7 antibiotic. EMBO J, 2009. 28(13): p. 1953;1964.
119. Severinov, K. and S.K. Nair, Microcin C: biosynthesis and mechanisms of bacterial resistance. Future Microbiol, 2012. 7(2): p. 281−289.
120. Novikova, M., et al., The Escherichia coli Yej Transporter Is Required for the Uptake of Translation Inhibitor Microcin C. J Bacteriol, 2007. 189(22): p. 8361−8365.
121. Eswarappa, S.M., et al., The yejABEF operon of Salmonella confers resistance to antimicrobial peptides and contributes to its virulence. Microbiology, 2008.154(2): p. 666−678.
122. Kazakov, T., et al., Escherichia coli Peptidase A, B, or N Can Process Translation Inhibitor Microcin C. J Bacteriol, 2008. 190(7): p. 2607−2610.
123. Metlitskaya, A., et al., Aspartyl-tRNA Synthetase Is the Target of Peptide Nucleotide Antibiotic Microcin C. Journal of Biological Chemistry, 2006. 281(26): p. 18 033−18 042.
124. Novoa, M.A., et al., Cloning and mapping of the genetic determinants for microcin C7 production and immunity. J Bacterid, 1986.168(3): p. 1384−1391.
125. Novikova, M., et al., MccE Provides Resistance to Protein Synthesis Inhibitor Microcin C by Acetylating the Processed Form of the Antibiotic. Journal of Biological Chemistry, 2010. 285(17): p. 12 662−12 669.
126. Agarwal, V., et al., Structural Basis for Microcin C7 Inactivation by the MccE Acetyltransferase. Journal of Biological Chemistry, 2011. 286(24): p. 21 295−21 303.
127. Fomenko, D., A. Veselovskii, and I. Khmel, Regulation of microcin C51 operon expression: the role of global regulators of transcription. Res Microbiol, 2001.152(5): p. 469−479.
128. Moreno, F., et al., The regulation of microcin B, C and J operons. Biochimie, 2002. 84(5−6): p.
129. Severinov, K., et al., Low-molecular-weight post-translationally modified microcins. Mol Microbiol, 2007. 65(6): p. 1380−1394.
130. Forrest, A.K., et al., Aminoalkyl adenylate and aminoacyl sulfamate intermediate analogues differing greatly in affinity for their cognate Staphylococcus aureus aminoacyl tRNA synthetases. Bioorg Med Chem Lett, 2000. 10(16): p. 1871−4.
131. Belrhali, H., et al., Crystal structures at 2.5 angstrom resolution of seryl-tRNA synthetase complexed with two analogs of seryl adenylate. Science, 1994. 263(5152): p. 1432−1436.
132. Cisar, J.S., et al., Exploiting Ligand Conformation in Selective Inhibition of Non-Ribosomal Peptide Synthetase Amino Acid Adenylation with Designed Macrocyclic Small Molecules. J Am Chem Soc, 2007. 129(25): p. 7752−7753.
133. Schimmel, P., J. Tao, and J. Hill, Aminoacyl tRNA synthetases as targets for new anti-infectives. The FASEB Journal, 1998. 12(15): p. 1599−1609.
134. Ubukata, M.O., H.- Isodo, K., Synthesis and biological activity of nucleoside antibiotics, ascamycin and its amino acid analogs. Nucleic Acids Symp Ser, 1985(16): p. 81−3.
135. Van de Vijver, P., et al., Antibacterial 5 P., et al., 1.,): p. 81−3.adenosines. Bioorganic &Medicinal Chemistry, 2009.17(1): p. 260−269.
136. Van de Vijver, P., et al., Synthetic Microcin C Analogs Targeting Different Aminoacyl-tRNA Synthetases. J Bacterid, 2009.191(20): p. 6273−6280.
137. Vondenhoff, G.H.M., et al., Extended targeting potential and improved synthesis of Microcin C analogs as antibacterials. Bioorganic &Medicinal Chemistry, 2011. 19(18): p. 5462−5467.
138. Vondenhoff, G.H.M., et al., Characterization of Peptide Chain Length and Constituency Requirements for YejABEF-Mediated Uptake of Microcin C Analogues. J Bacteriol, 2011. 193(14): p.
139. Korza, H.J. and M. Bochtler, Pseudomonas aeruginosa LD-Carboxypeptidase, a Serine Peptidase with a Ser-His-Glu Triad and a Nucleophilic Elbow. Journal of Biological Chemistry, 2005. 280(49): p. 40 802−40 812.
140. Page, M. and E. Di Cera, Serine peptidases: Classification, structure and function. Cellular and Molecular Life Sciences, 2008. 65(7): p. 1220−1236.
141. Schechter, I. and A. Berger, On the size of the active site in proteases. I. Papain. Biochem Biophys Res Commun, 1967. 27(2): p. 157−62.521.529.3618−3623.