Регуляция экспрессии генов системы рестрикции-модификации типа II Eco29kl
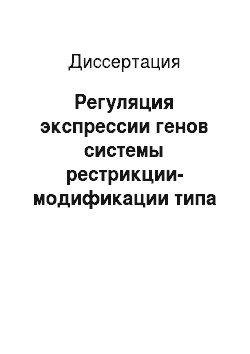
Выявление механизмов регуляции экспрессии генов является задачей фундаментальных исследований в молекулярной биологии. Однако результаты этих исследований позволяют применять накопленные знания на практике. Регуляторные механизмы обнаруженные в системах рестрикции-модификации, могут с успехом применяться для создания искусственных молекулярных переключателей генной экспрессии. В частности… Читать ещё >
Содержание
- СПИСОК СОКРАЩЕНИЙ
- Глава 1. ОБЗОР ЛИТЕРАТУРЫ
- 1. 1. Системы рестрикции-модификации у прокариот
- 1. 1. 1. Явление рестрикции-модификации
- 1. 1. 2. Типы систем рестрикции-модификации
- 1. 1. 3. Биологическая роль систем рестрикции-модификации
- 1. 1. 4. Структурная организация систем рестрикции-модификации типа II
- 1. 1. 5. Регуляция экспрессии генов систем рестрикции-модификации типа II
- 1. 1. 5. 1. Регуляция экспрессии генов систем рестрикции-модификации типа II метилтрансферазой
- 1. 1. 5. 2. Регуляция экспрессии генов систем рестрикции-модификации типа II С-белками
- 1. 1. 5. 3. Регуляция экспрессии генов «линейных» систем рестрикции-модификации типа II
- 1. 1. Системы рестрикции-модификации у прокариот
- 1. 2. Регуляция экспрессии генов антисмысловыми РНК
- 1. 2. 1. Биологические функции малых антисмысловых РНК
- 1. 2. 2. Характеристика цис-кодируемых антисмысловых РНК
- 1. 2. 3. Механизмы действия цис-кодируемых антисмысловых РНК
- 1. 2. 4. Различия между цис-кодируемыми и транс-кодируемыми асРНК
- 2. 1. Материалы и реактивы
- 2. 1. 1. Штаммы бактерий и плазмидные вектора
- 2. 1. 2. Среды и основные буферы
- 2. 1. 3. Материалы и реактивы
- 2. 2. Методы исследования
- 2. 2. 1. Получение бактериальной биомассы
- 2. 2. 2. Выделение плазмидной ДНК
- 2. 2. 3. Выделение тотальной РНК
- 2. 2. 4. Препаративное выделение фрагмента ДНК
- 2. 2. 5. Получение препарата фага A. vir в высоком титре
- 2. 2. 6. Получение компетентных клеток Е. coli и их трансформация
- 2. 2. 7. Анализ рекомбинантных клонов
- 2. 2. 8. Полимеразная цепная реакция (ПЦР)
- 2. 2. 9. Мечение 5'-конца ДНК полинуклеотидкиназой фагаТ
- 2. 2. 10. Определение и анализ первичной последовательности ДНК
- 2. 2. 11. Цитирование фрагментов ДНК
- 2. 2. 12. Реакция транскрипции in vitro
- 2. 2. 13. КМ11О4 (перманганатный) фут-принтинг
- 2. 2. 14. Реакция удлинения праймера
- 2. 2. 15. ПЦР с детекцией продуктов в режиме реального времени
- 2. 2. 16. Электрофорез нуклеиновых кислот
- 2. 2. 17. Northern-гибридизация
- 2. 2. 18. Определение (3-галактозидазной активности
- 2. 2. 19. Сайт-направленный мутагенез
- 3. 1. Структурная организация генов системы рестрикции-модификации типа II Eco29kI
- 3. 2. Промоторы гена эндонуклеазы рестрикции системы рестрикции-модификации типа II Eco29kI
- 3. 3. Промотор гена метилтрансферазы системы рестрикции-модификации типа II Eco29kI
- 3. 4. Система рестрикции-модификации типа II Eco29kI кодирует два типа мРНК
- 3. 5. Антисенс-промоторы системы рестрикции-модификации типа II Eco29kI
- 3. 6. Влияние транскрипции с антисенс-промоторов на транскрипцию гена эндонуклеазы рестрикции eco29kIR
- 3. 7. Влияние активности антисенс-промоторов на экспрессию гена эндонуклеазы рестрикции eco29kIR
- 3. 8. Активность антисенс-промоторов вызывает деградацию бицистронной мРНК
- 3. 9. Модель регуляции экспрессии генов системы рестрикции-модификации типа II Eco29kI
Регуляция экспрессии генов системы рестрикции-модификации типа II Eco29kl (реферат, курсовая, диплом, контрольная)
Крупнейшая часть живых организмов в биосфере представлена бактериями, поэтому большая часть генетической информациибактериальная (Whitman et al., 1998). Главной основой эволюционного развития бактерий является горизонтальный перенос генов. (Holmes et al., 2003; Lawrence, 2001; Jain, 2002; Gogarten, 2002). Среди процессов, контролирующих перенос генов, одну из центральных ролей играет действие систем рестрикции-модификации.
К настоящему времени открыто несколько тысяч систем рестрикции-модификации в бактериях и архебактериях (Roberts et al., 2007). Наибольший интерес вызвало изучение систем рестрикции-модификации типа II, которые состоят из двух ферментов, узнающих одинаковую последовательность ДНК, но имеющих разное действие — эндонуклеазы рестрикции и метилтрансферазы. Эндонуклеаза вносит двухцепочечный разрыв в немодифицированной ДНК (например, вирусной ДНК во время инфицирования бактериальной клетки бактериофагом), а метилтрансфераза защищает хозяйскую ДНК от действия эндонуклеазы рестрикции, модифицируя основания ДНК в сайте узнавания. Таким образом, система рестрикции-модификации образует некую примитивную иммунную систему в бактериальной клетке. Однако системы рестрикции-модификации обеспечивают не только сохранность генетической информации бактериальной клетки-хозяина, но и обеспечивают горизонтальный перенос генов, что обеспечивает бактериальной популяции постоянное эволюционирование и адаптацию к новым экологическим условиям. Несомненно, что интерес к изучению систем рестрикции-модификации типа II был обусловлен использованием эндонуклеаз рестрикции этого типа в качестве одного из мощнейших инструментов научных исследований в молекулярной биологии. Но и кроме прикладного аспекта, системы рестрикции-модификации типа II представляют собой хорошую модель для изучения взаимодействия биологических макромолекул. Благодаря разнообразию структурной организации таких систем, они очень удобны для исследования регуляции экспрессии генов, так как экспрессия обоих ферментов должна быть строго регулирована, чтобы сохранять баланс между этими антагонистичными ферментативными активностями в бактериальной клетке. Поскольку исследование молекулярных механизмов регуляции экспрессии генов является одной из фундаментальных задач молекулярной биологии, то использование систем рестрикции-модификации типа II в качестве модельных объектов трудно переоценить.
До настоящего времени основное внимание уделялось изучению регуляции систем рестрикции-модификации типа II на уровне транскрипции, где основными регуляторами экспрессии генов являются метилтрансферазы или С-белки. Однако оставалась группа системы рестрикции-модификации типа II, о регуляции которых известно крайне мало. В эту группу входит система рестрикции-модификации Eco29kI (СРМ Eco29kI), регуляция которой была исследована в данной работе.
Цели и задачи исследования.
Целью настоящей работы являлось исследование регуляции экспрессии генов СРМ Eco29kI. Для достижения указанной цели последовательно ставились следующие задачи:
— исследовать организацию регуляторных элементов в СРМ Eco29kI;
— определить роль каждого из найденных регуляторных элементов в регуляции экспрессии генов СРМ Eco29kI;
— предложить модель регуляции СРМ Eco29kI;
Данная работа выполнена в лаборатории молекулярной микробиологии Учреждения Российской академии наук Института биохимии и физиологии микроорганизмов имени Г. К. Скрябина РАН и в лаборатории проф. Северинова К. В. института микробиологии Ваксмана, университет Ратгерс штата Нью-Джерси, США.
Научная новизна работы.
Впервые получены данные о регуляции экспрессии генов СРМ Eco29kI, которая относится к группе так называемых «линейных» систем рестрикции-модификации типа II.
Продемонстрировано, что перед геном эндонуклеазы рестрикции находятся два промотора, с которых происходит синтез бицистронной мРНК и она кодирует оба фермента СРМ Eco29kI. Установлено, что в структурной части гена эндонуклеазы находится дополнительный промотор гена метилтрансферазы, с которого транскрибируется моноцистронная мРНК, кодирующая только метилтрансферазу. Показано, что в структурной части гена эндонуклеазы находятся два промотора имеющие обратную ориентацию. Транскрипция с этих промоторов происходит в антисенс-направлении. Показано негативное влияние на экспрессию гена эндонуклеазы этих антисенс-промоторов на пост-транскрипционном уровне.
Практическое значение работы.
Выявление механизмов регуляции экспрессии генов является задачей фундаментальных исследований в молекулярной биологии. Однако результаты этих исследований позволяют применять накопленные знания на практике. Регуляторные механизмы обнаруженные в системах рестрикции-модификации, могут с успехом применяться для создания искусственных молекулярных переключателей генной экспрессии. В частности, природный механизм подавления экспрессии токсичного для бактериальной клетки гена эндонуклеазы в СРМ Eco29kI, может быть применен для создания искусственных антисенс-РНК-ингибиторов генной экспрессии. Это позволяет применять данный способ регуляции экспрессии генов, как в научных исследованиях, так и в практической деятельности.
ВЫВОДЫ:
1. Охарактеризованы два промотора гена ЭР и отдельный промотор гена МТ. С них транскрибируются мРНК двух типов: бицистронная и моноцистронная.
2. В структурной части гена ЭР обнаружены два промотора, транскрипция с которых конвергентна транскрипции гена ЭР.
3. Показано, что антисенс-промоторы не оказывают влияния на транскрипцию гена ЭР.
4. Антисенс-промоторы снижают экспрессию гена ЭР на посттранскрипционном уровне.
5. На основе полученных результатов представлена модель регуляции СРМ Eco29kI.
Список литературы
- Alvarez М., Chater К. and Rodicio R. (1993) Complex transcription of an operon encoding the Sail restriction-modification system of Streptomyces albus G. Mol. Microbiology 8, 243−252.
- Anderson, D.G., Churchill, J.J. & Kowalczykowski, S.C.(1999) A single mutation, RecB (D1080A), eliminates RecA protein loading but not chi recognition by RecBCD enzyme. JBC 274, 27 139−27 144.
- Arber W. (1965) Host specificity of DNA produced by Escherichia coli. V. The role of methionine in the production of host specificity. J. Mol. Biol. 11,247−256.
- Arber W., and Dussoix D. (1962) Host specificity of DNA produced by Escherichia coli. I. Host controlled modification of bacteriophage lambda. J. Mol. Biol. 5, 18−36.
- Arber, W. (2000) Genetic variation: molecular mechanisms and impact on microbial evolution. FEMS Microbiol. Rev. 24, 1−7.
- Arthur D., Ghetu A., Gubbins M., Edwards R., Frost L., Glover J. (2003) FinO is an RNA chaperone that facilitates sense-antisense RNA interactions. EMBO J 22, 6346−6355.
- Asano K., Mizobuchi K. (2000) Structural analysis of late intermediate complex formed between plasmid Collb-P9 Inc RNA and its target RNA. How does a single antisense RNA repress translation of two genes at different rates? JBC 275, 1269−1274.
- Asano K., Niimi Т., Yokoyama S., Mizobuchi K. (1998) Structural basis for binding of the plasmid Collb-P9 antisense Inc RNA to its target RNA with the 5'rUUGGCG3' motif in the loop sequence. JBC 273, 11 826−11 838.
- Ban, C. & Yang, W. (1998) Structural basis for MutH activation in E. coli mismatch repair and relationship of MutH to restriction endonucleases. EMBO J. 17, 1526−1534.
- Barcus, V.A. & Murray, N.E. Barriers to recombination: restriction.(Cambridge University Press, Cambridge, 1995)
- Bart A., Dankert J., and van der Ende A. (1999) Operator sequences for the regulatory proteins of restriction modification systems. Mol. Microbiol. 31, 1277−1278.
- Beletskaya I. V., Zakharova M. V., Shlyapnikov M. G., Semenova L. M., and Solonin A. S. (2000) DNA methylation at the CfrBI site is involved in expression control in the CfrBI restriction-modification system. Nucleic Acids Res. 28,3817−3822.
- Bertani G., and Weigle J. J. (1953) Host controlled variation in bacterial viruses. J. Bacterid. 65, 113−121.
- Bickle, T.A. & Kruger, D.H. (1993) Biology of DNA restriction. Microbiol.Rev. 57, 434−450.
- Blumenthal, R.M. and Cheng, X. Restriction-modification systems, in Modern Microbial Genetics (eds. Yasbin, R. & Streips, U.) 177−226 (Wiley-Liss, New York, 2002).
- Bogdanova E, Djordjevic M, Papapanagiotou I, Heyduk T, Kneale G, Severinov K. (2008) Transcription regulation of the type II restriction-modification system. Nucleic Acids Res. 36, 1429−1442.
- Brantl S, Wagner E. (2000) Antisense RNA-mediated transcriptional attenuation: an in vitro study of plasmid pT181. Mol Microbiol 35, 14 691 482.
- Brantl S. (2002) Antisense-RNA regulation and RNA interference. Biochim. Biophys. Acta 1575, 15−25.
- Brantl S. (2007) Regulatory mechanisms employed by c/s-encoded antisense RNAs. Current Opinion in Microbiology 10, 102−109.
- Brantl S., Wagner E. (1994) Antisense RNA-mediated transcriptional attenuation occurs faster than stable antisense/target RNA pairing: an in vitro study of plasmid pIP501. EMBO J 13, 3599−3607.
- Brantl S., Wagner E. (1996) An unusually long-lived antisense RNA in plasmid copy number control: in vivo RNAs encoded by the streptococcal plasmid pIP501. JMB 255, 275−288.
- Brennan R.G., Roderick S.L., Takeda Y. and Matthews B.W. (1990) Protein-DNA conformational changes in the crystal structure of a lambda Cro-operator complex. Proc. Natl. Acad. Sci. USA 87, 8165−8169.
- Brenner M., Tomizawa J. (1991) Quantitation of ColEl-encoded replication elements. PNAS 88, 405−409.
- Brussow, H. (2007) Bacteria between protists and phages: from antipredation strategies to the evolution of pathogenicity. Mol.Microbiol. 65, 583−589.
- Butler D., and Fitzgerald G. F. (2001) Transcriptional analysis and regulation of expression of the ScrFI restriction-modification system of Lactococcus lactis subsp. cremoris UC503. J. Bacteriol. 183, 4668−4673.
- Campbell, A.M. in Escherichia coli and Salmonella: cellular and molecular biology. 2041−2046 (ASM Press, Washington, D.C. 1996)
- Carlson, K. & Kosturko, L.D. (1998) Endonuclease II of coliphage T4: a recombinase disguised as a restriction endonuclease? Mol. Microbiol 27, 671−676.
- Casjens, S. (2003) Prophages and bacterial genomics: what have we learned so far? Mol.Microbiol. 49, 277−300.
- Cesnaviciene E., Mitkaite G., Stankevicius K., Janulaitis A., and Lubys A. (2003) Espl396I restriction-modification system: structural organization and mode of regulation. Nucleic Acids Res. 31, 743−749.
- Chibani-Chennoufi, S., Bruttin, A., Dillmann, M.L. & Brussow, H. (2004) Phage-host interaction: an ecological perspective. JB 186, 3677−3686.
- Christensen L. L., and Josephsen J. (2004) The methyltransferase from the LlaDII restriction-modification system influences the level of expression of its own gene. J. Bacterid. 186, 287−295.
- Churchill, J.J., Anderson, D.G. & Kowalczykowski, S.C. (1999) The RecBC enzyme loads RecA protein onto ssDNA asymmetrically and independently of chi, resulting in constitutive recombination activation. Genes Dev. 13,901−911.
- Dawkins R. The Selfish Gene. (Oxford University Press, Oxford. 1989)
- Duhring U., Axmann I., Hess W., Wilde A. (2006) An internal antisense RNA regulates expression of the photosynthesis gene isiA. PNAS 103, 7054−7058.
- Dussoix D., and W. Arber. (1962) Host specificity of DNA produced by Eschenichia coli. II. Control over acceptance of DNA from infecting phage lambda. J. Mol. Biol. 5, 37−49.
- Eguchi Y., Ithoh Т., Tomizawa J. (1991) Antisense RNA. Annu Rev Biochem 60, 631−652.
- Engelberg-Kulka H. and Glaser G. (1999) Addiction modules and programmed cell death and antideath in bacterial cultures Annu. Rev. Microbiol. 53,43−70.
- Feinstein, S.I. & Low, K.B. (1982) Zygotic induction of the rac locus can cause cell death in E. coli. Mol.Gen.Genet. 187, 231−235.
- Fire A. et al. (1998) Potent and specific genetic interference by double-stranded RNA in Caenorhabditis elegans. Nature 391, 806−811.
- Franch Т., Petersen M., Wagner E.G.H., Jacobsen J., Gerdes K. (1999) Antisense RNA regulation in prokaryotes: rapid RNA/RNA interaction facilitated by a general U-turn-loop structure. JMB 294, 1115−1125.
- Gerdes K., Wagner E. (2007) RNA antitoxins. Curr Opin Microbiol 10, 117−124.
- Gogarten, J. P., Doolittle, W.F. & Lawrence, J.G. (2002) Prokaryotic evolution in light of gene transfer. Mol. Biol. Evol. 19, 2226−2238.
- Greenfield Т., Franch Т., Gerdes K" Weaver K. (2001) Antisense RNA regulation of the par post-segregational killing system: structural analysis and mechanism of binding of the antisense RNA, RNAII and its target RNAI. Mol Microbiol 42, 527−537.
- Gubbins M., Arthur D., Ghetu A., Glover J., Frost L. (2003) Characterizing the structural features of RNA/RNA interactions of the F-plasmid FinOP fertility inhibition system. JBC 278, 27 663−27 671.
- Handa N., Ichige A., Kusano K. and Kobayashi I. (2000) Cellular responses to postsegregational killing by restriction-modification genes. J. Bacterid. 182,2218−2229.
- Heidrich N., Brantl S. (2003) Antisense-RNA mediated transcriptional attenuation: Importance of a U-turn loop structure in the target RNA of plasmid pIP501 for efficient inhibition by the antisense-RNA. JMB 333, 917−929.
- Heidrich N., Brantl S. (2007) Antisense-RNA mediated transcriptional attenuation in plasmid pIP501: the simultaneous interaction between two complementary loop pairs is required for efficient inhibition by antisense RNA. Microbiol 153,420−427.
- Heidrich N" Chinali A., Gerth U" Brantl S. (2006) The small untranslated RNA SRI from the B. subtil is genome is involved in the regulation of arginine catabolism. Mol Microbiol 62, 520−536.
- Hjalt Т., Wagner E.G.H. (1992) The effect of loop size in antisense and target RNAs on the efficiency of antisense RNA control. NAR 20, 67 236 732.
- Hjalt Т., Wagner E.G.H. (1995) Bulged-out nucleotides in an antisense RNA are required for rapid target RNA binding in vitro and inhibition in vivo. NAR 23, 580−587.
- Holmes, A.J., Gillings, M.R., Nield, B.S., Mabbutt, B.C., Nevalainen, K.M. & Stokes, H.W. (2003) The gene cassette metagenome is a basic resource for bacterial genome evolution. Environ. Microbiol. 5, 383−394.
- Ives C.L., Nathan P.D., Brooks J.E. (1992) Regulation of the BamHI restriction-modification system by a small intergenic open reading frame, bamHIC, in both Escherichia coli and Bacillus subtilis. J. Bacteriol. 174, 7194−7201.
- Ives C.L., Sohail A., Brooks J.E. (1995) The regulatory С Proteins from different restriction-modification systems can cross-complement. J. Bacteriol. 6313−6315.
- Jain, R., Rivera, M.C., Moore, J.E. & Lake, J.A. (2002) Horizontal gene transfer in microbial genome evolution. Theor. Popul. Biol. 61, 489−495.
- Jerome L., van Biesen Т., Frost L. (1999) Degradation of FinP antisense RNA from F-like plasmids: the RNA binding protein, FinO, protects FinP from endoribonuclease E. JMB 285, 1457−1473.
- Jordan S.R. and Pabo C.O. (1988) Structure of the lambda complex at 2.5 A resolution: details of the repressor-operator interactions. Science 242, 893−899.
- Kessler C., Manta V. (1990) Specificity of restriction endonucleases and DNA modification methyltransferases a review (Edition 3). Gene 92, 1248.
- Kita К., ICotani H., Sugisaki H., and Takanami M. (1989) The Fokl restriction-modification system. I. Organization and nucleotide sequences of the restriction and modification genes. J. Biol. Chem. 264, 5751−5756.
- Knowle D., Lintner R., Touma Y. M., Blumenthal R. M. (2005) Nature of promoter activated by C. PvuII, an unusual regulatory protein conserved among restriction-modification systems. J. Bacteriol. 187, 488−497.
- Kobayashi I. (1998) Selfishness and death: raison d’etre of restriction, recombination and mitochondria. Trends. Genet. 14, 368−74.
- Kolb F., Westhof E., Ehresman C., Ehresman В., Wagner E., Romby P. (2001) Bulged residues promote the progression of a loop-loop interaction to a stable and inhibitory antisense-target RNA complex. NAR 29, 3145−3153.
- Krinke L., Wulff D. (1987) OOP RNA, produced from multicopy plasmids, inhibits ell gene expression through an RNase Ill-dependent mechanism. Genes Dev 1, 1005−1012.
- Krinke L., Wulff D. (1990) RNase Ill-dependent hydrolysis of cll-0 gene mRNA by OOP antisense RNA. Genes Dev 4, 2223−2233.
- Kruger, D.H. & Bickle, T.A. (1983) Bacteriophage survival: multiple mechanisms for avoiding the deoxyribonucleic acid restriction systems of their hosts. Microbiol. Rev. 47, 345−360.
- Kuzminov, A., Schabtach, E & Stahl, F.W. (1994) Chi sites in combination with RecA protein increase the survival of linear DNA in E. coli by inactivating ExoV activity of RecBCD nuclease. EMBO J. 13, 2764−2776.
- Lao, P.J. & Forsdyke, D.R. (2000) Crossover hot-spot instigator (Chi) sequences in E. coli occupy distinct recombination/transcription islands. Gene 243, 47−57.
- Lawrence, J.G. (2001) Catalyzing bacterial speciation: correlating lateral transfer with genetic headroom. Syst. Biol. 50, 479−496.
- Liu Y. and Kobayashi I. (2007) Negative regulation of the EcoRI restriction enzyme gene is associated with intragenic reverse promoters. JB 189, 6928−6935.
- Lubys A., and Janulaitis A. (1995) Cloning and analysis of the plasmid-borne genes encoding the Bsp6I restriction and modification enzymes. Gene 157, 25−29.
- Lubys A., Jurenaite S., Janulaitis A. (1999) Structural organization of the plasmid-borne restriction-modification system type II Kpn2I from Klebsiella Pneumoniae RLF2. Nucleic Acids Res. 27, 4228−4234.
- Lubys A., Menkevicius S., Timinskas A., Butkus V. and Janulaitis A. (1994) Cloning and analysis of translational control for genes encoding the Cfr9I restriction-modification system. Gene 141, 85−89.
- Lurlia S. E., and Human M. L. (1952) A nonhereditaxy, host-induced variation of bacterial viruses. J. Bacteriol. 64, 557−569.
- McGeehan J.E., Papapanagiotou I., Streeter S.D., Kneale G.G. (2006) Cooperative binding of the C. Ahdl controller protein to the C/R promoter and its role in endonuclease gene expression. J. Mol. Biol. 358, 523−531.
- McGeehan J.E., Streeter S.D., Papapanagiotou I., Fox G.C. and Kneale G.G. (2005) High-resolution crystal structure of the restriction-modification controller protein C. Ahdl from Aeromonas hydrophila. J. Mol. Biol. 346, 689−701.
- Milkman, R., Raleigh, E.A., McKane, M., Cryderman, D., Bilodeau, P. & McWeeny, K. (1999) Molecular evolution of the E. coli chromosome. V. Recombination patterns among strains of diverse origin. Genetics 153, 539 554.
- Moll I., Grill S., Gualerzi С. O., and Blasi U. (2002) Leaderless mRNAs in bacteria: surprises in ribosomal recruitment and translational control. Mol. Microbiol. 43,239−246.
- Naito Т., Kusano K. and Kobayashi I. (1995) Selfish behavior of restriction-modification systems. Science 267, 897−899.
- Nakayama Y. and Kobayashi I. (1998) Restriction-modification gene complexes as selfish gene entities: roles of a regulatory system in theirestablishment, maintenance, and apoptotic mutual exclusion. Proc. Natl. Acad. Sci. USA 95, 6442−6447.
- O' Connor C. and Humphreys G. (1982) Expression of the EcoRI restriction-modification system and the construction of positive-selection cloning vectors. Gene 20, 219−229.
- O’Driscoll J., Glynn F., Cahalane 0., O’Connell-Motherway M., Fitzgerald G.F., and Van Sinderen D. (2004) Lactococcal plasmid pNP40 encodes a novel, temperature-sensitive restriction-modification system. Appl. Environ. Microbiol. 70, 5546−5556.
- O’Driscoll J., Fitzgerald G.F., van Sinderen D. (2005) A dichotomous epigenetic mechanism governs expression of the LlaJI restriction/modification system. Mol. Microbiol. 57, 1532−1544.
- Opdyke J., Kang J., Storz G. (2004) GadY, a small RNA regulator of acid response genes in Escherichia coli. JB 186, 6698−6705.
- Pertzev A., Ruban N., Zakharova M., Beletskaya I., Petrov S., Kravetz A., Solonin A. (1991) Eco29kI, a novel plasmid encoded restriction endonuclease from Escherichia coli. Nucleic Acids Res. 20
- Petrusyte M., Bitinaite J., Menkevicius S., Klimasauskas S., Butkus V., Janulaitis A. (1988) Restriction endonucleases of a new type. Gene 74, 8991.
- Pingoud A. M. Restriction endonucleases // Nucleic Acids and Molecular Biology (ed. Hans Joachim Gross) V. 14. (Springer-Verlag Berlin, Heidelberg, 2004)
- Posfai J., Bhagwat A.S., Posfai G. and Roberts R.J. (1989) Predictive motifs derived from cytosine methyltransferases. Nucleic Acids Res. 17, 2421−2435.
- Price, C. & Bickle, T.A. (1986) A possible role for DNA restriction in bacterial evolution. Microbiol. Sci. 3, 296−299.
- Rak B, von Reutern M (1984) Insertion element IS5 contains a third gene. EMBO J. 3,807−811.
- Rasmussen A., Eriksen M., Gilany K., Udesen C., Franch Т., Petersen C., Valentin-Hansen P. (2006) Regulation of ompA mRNA stability: the role of a small regulatory RNA in growth phase-dependent control. Mol Microbiol 58, 1421−1429.
- Roberts, R.J., Vincze, Т., Posfai, J. & Macelis, D. (2007) REBASE -enzymes and genes for DNA restriction and modification. NAR 35, D269−270.
- Sarkar G. and Sommer S. (1990) The «megaprimer» method of site-directed mutagenesis. BioTechniques 8, 404−407.
- Sawaya M.R., Zhu Z" Mersha F., Chan S.H., Dabur R" Xu S.Y., Balendiran G.K. (2005) Crystal structure of the restriction-modification system control element C. Bcll and mapping of its binding site. Structure 13, 1837−1847.
- Semenova E., Minakhin L., Bogdanova E., Nagornykh M., Vasilov A., Heyduk Т., Solonin A., Zakharova M., Severinov K. (2005) Transcription regulation of the EcoRV restriction-modification system. Nucleic Acids Res. 33,6942−6951.
- Shilov I., Tashlitsky V., Khodoun M., Vasil’ev S., Alekseev Y., Kuzubov A., Kubareva E., Kaiyagina A. (1998) DNA-methyltransferase SsoII interaction with own promoter region binding site. Nucleic Acids Res. 26, 2659−64.
- Silvaggi J., Perkins J., Losick R. (2005) Small untranslated RNA antitoxin in Bacillus subtilis. JB 187,6641−6650.
- Som S., and Friedman S. (1994) Regulation of EcoRII methyltransferase: effect of mutations on gene expression and in vitro binding to the promoter region. Nucleic Acids Res. 22, 5347−5353.
- Som S., and Friedman S. (1997) Characterization of the intergenic region which regulates the Mspl restriction-modification system. J. Bacteriol. 179, 964−967.
- Spanjaard R.A. and Van Duin J. (1989) Translation reinitiation in the presence and absence of a Shine and Dalgarno sequence. NAR 17, 55 015 507.
- Streeter S.D., Papapanagiotou I., McGeehan J.E. and Kneale G.G. (2004) DNA footprinting and biophysical characterization of the controller protein C. Ahdl suggests the basis of a genetic switch. Nucleic Acids Res. 32, 644 553.
- Suzuki M. and Yagi N. (1994) DNA recognition code of transcription factors in the helix-turn-helix, probe helix, hormone receptor, and zinc finger families. Proc. Natl. Acad. Sci. USA 91, 12 357−12 361.
- Suzuki M., Brenner S.E., Gerstein M. and Yagi N. (1995) DNA recognition code of transcription factors. Protein Eng. 8, 319−328.
- Tao T. and Blumenthal R.M. (1992) Sequence and characterization of pvuIIR, the PvuII endonuclease gene, and of pvuIIC, its regulatory gene. J. Bacterid. 174,3395−3398.
- Tao Т., Bourne J.C., Blumenthal R.M. (1991) A familiy of regulatory genes associated with type II restriction-modification systems. J. Bacterid. 174,1367−1375.
- Trautner, T.A., Pawlek, В., Behrens, B. & Willert, J. (1996) Exact size and organization of DNA target-recognizing domains of multispecific DNA-(cytosine-C5)-methyltransferases. EMBO J. 15, 1434−1442.
- Vijesurier R. M" Carlock L., Blumenthal R. M., and Dunbar J. C. (2000) Role and mechanism of action of C. PvuII, a regulatory protein conserved among restriction-modification systems. J. Bacterid. 182, 477−487.
- Vogel J., В artels V., Tang Т., Churakov G" Slagter-Jager J., Huttenhofer A., Wagner E. (2003) RNomics in Escherichia coli detects new sRNA species and indicates parallel transcriptional output in bacteria. NAR 31, 6435−6443.
- Wagner E.G.H. and Simons R.W. (1994) Antisense RNA control in bacteria, phages and plasmids. Annu. Rev. Microbiol. 48, 713−742.
- Wagner L., Gesteland R., Dayhuff T. and Weiss R. (1994) An efficient Shine-Dalgarno sequence but not translation is necessary for lacZ mRNA stability in Escherichia coli. JB 176, 1683−1688
- Waldbeser L., Chen Q., Crosa J. (1995) Antisense RNA regulation of the fatB iron transport protein gene in Vibrio anguillarum. Mol Microbiol 17, 747−756.
- Wang, J., Chen, R. & Julin, D.A. (2000) A single nuclease active site of the E. coli RecBCD enzyme catalyzes single-stranded DNA degradation in both directions. JBC 275, 507−513.
- Weaver K. (2007) Emerging plasmid-encoded antisense RNA regulated systems. Curr Opin Microbiol 10, 110−116.
- Weinbauer, M.G. (2004) Ecology of prokaryotic viruses. FEMS Microbiol. Rev. 28, 127−181.
- Weitz, J.S., Hartman, H. & Levin, S.A. (2005) PNAS 102, 9535−9540.
- Whitman, W.B., Coleman, D.C. & Wiebe, W.J. (1998) Prokaiyotes: the unseen majority. PNAS 95, 6578−6583.
- Will W., Frost L. (2006) Hfq is a regulator of F-plasmid TraJ and TraM synthesis in E. coli. JB 188, 124−131.
- Wilson G.G. (1991) Organization of restriction-modification systems. Nucleic Acids Res. 19, 2539−2565.
- Yang C.C., Topal M.D. (1992) Nonidentical DNA binding sites of endonuclease Nael recognize different families of sequences flanking the recognition sate. Biochemistry 31, 9657−9664.
- Zakharova M., Beletskaya I., Kravetz A., Pertzev A., Mayorov S., Shlyapnikov M. and Solonin A. (1998) Cloning and sequence analysis of the plasmid-bome genes encoding the Eco29kI restriction and modification enzymes. Gene 208, 177−182.
- Zakharova M., Minakhin L., Solonin A., and Severinov K. (2004) Regulation of RNA polymerase promoter selectivity by covalent modification of DNA. J. Mol. Biol. 335, 103−111.
- Zamore P.D. (2001) RNA interference: listening to the sound of silence. Nat Struct. Biol. 8, 746−750.
- Zhang A., Wassarman K., Rosenow C., Tjaden В., Storz G., Gottesman S. (2003) Global analysis of small RNA and mRNA targets of Hfq. Mol Microbiol 50, 1111−1124.
- Zheleznaya L.A., Kainov D.E., Yunusova A.K., Matvienko N.I. (2003) Regulatory С protein of the EcoRV modification-restriction system. Biochemistry (Mosc). 68, 125−132.1. БЛАГОДАРНОСТИ
- Я искренне благодарен своим научным руководителям к.б.н. Захаровой Марине Викторовне и д.б.н. Северинову Константину Викторовичу за руководство и помощь при выполнении данной работы.
- Я благодарен зав. лабораторией «Молекулярной микробиологии» к.б.н. Солонину Александру Сергеевичу за поддержку и понимание.